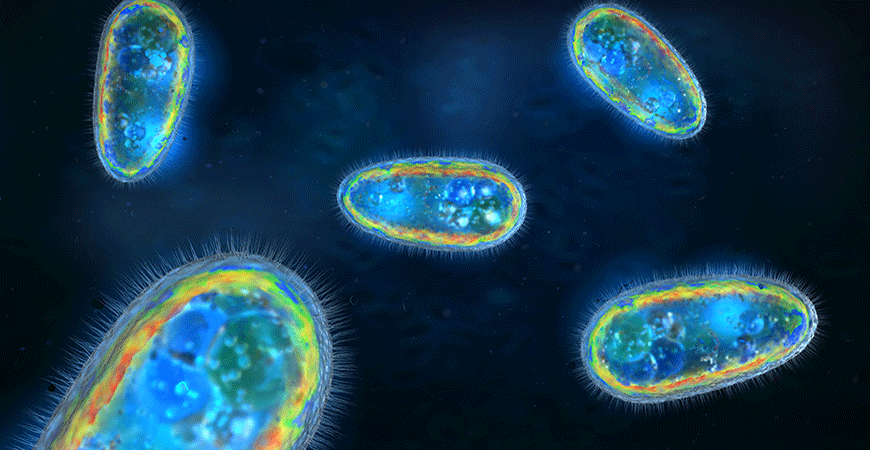
Theoretical physics Professor Ajay Gopinathan has been working over the past decade to model a submicroscopic mystery. Now, he and a team of colleagues have verified an important piece of the puzzle of how tiny, intrinsically twisted protein filaments responsible for repairing and growing cells know where to go to perform their function.
The work could someday enable scientists to control bacterial growth.
Bacteria come in a variety of sizes and shapes, including spheres, rods and spirals. Each one’s size and shape is determined by its rigid outer wall. Maintaining these shapes as the bacteria grow requires the coordinated growth of the cell walls in the right places at the right times.
Scientists know that tiny, twisted, protein filaments called MreB congregate in regions where growth needs to happen and direct the expansion. But knowing where growth is needed requires measuring the shape and size of the cell.
“How do these protein filaments recognize where the cell needs to grow or heal?” Gopinathan asked. “They need to sense the local curvature of the cell wall, but they are nanoscopic and the cell is so much bigger than they are.”
Gopinathan, chair of the Department of Physics, co-director of the NSF-CREST Center for Cellular and Biomolecular Machines (CCBM) and a member of the Health Sciences Research Institute, has been exploring the idea that a generic twisted filament can bind itself to the cell’s inner surface and untwist to “measure” the cell’s curvature.
He worked with CCBM computational project scientist David Quint and Professor Greg Grason from the University of Massachusetts at Amherst on developing a theory based on this idea. The team has published work over the years showing that the local shape of a surface determines the propensity for twisted filaments to untwist and bind there, as well as the preferred lengths and orientations of the bound filaments. This geometric selection can ensure that large classes of filaments bind and congregate only in regions of particular shapes.
As Gopinathan and his colleagues were publishing their findings, experts in bacterial growth, Professor K.C. Huang and postdoctoral researcher Handuo Shi, at Stanford University, worked at the atomic scale to characterize the twisting of several mutant forms of MreB at the molecular scale, and also measured their lengths, locations and orientations in live bacterial cells.
Realizing that the theory could provide a direct connection between the atomic- and cell-scale information, the two teams got together. The collaborators were able to theoretically predict the lengths and orientations of the MreB filaments and how they depended on the particular mutant’s filament twist. Their observations in live cells confirmed their predictions, validating the idea that the filament twist at the molecular scale could potentially control growth at the cell scale.
The researchers detailed their work in the journal Nature Communications recently.
“This work tells us that if we make small changes to the filaments, we can affect — or control — bacterial growth,” Gopinathan said. Such an understanding could pave the way for new antibacterial targets.
“Moving forward, our approach also provides the opportunity to design and engineer cell shape by directly tuning MreB properties or by creating new MreB twist mutants,” Shi said. Future steps include understanding the growth and motion of the filaments themselves in conjunction with the growing cell walls.
Gopinathan said the team plans to continue exploring these avenues.
“I anticipate a lot more progress in this exciting field at the intersection of physics and biology,” he said.
Lorena Anderson
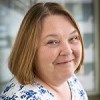
Senior Writer and Public Information Representative
Office: (209) 228-4406
Mobile: (209) 201-6255